Microscopic splits or breaks, known as microcracks, can occur within the cells or protective layers of a solar panel. These small, often invisible cracks significantly impact the solar panel's performance and durability. Microcracks can lead to reduced power output, a shortened lifespan, or even a complete halt in energy production. This article delves into the reasons behind the formation of microcracks in solar panels, methods for their detection, the consequences they bring, and how to select solar panels that are less prone to developing these cracks.
Contents:
- Why Are There Microcracks on My Solar Panels?
- What Damage Can Be Caused by Microcracks?
- How to Inspect for Microcracks During the Installation and Operation of Solar Panels?
- Choose the Right Solar Panels To Reduce Microcracks
Why Are There Microcracks on My Solar Panels?
Microcracks can emerge from a variety of sources, often related to manufacturing defects:
Production Flaws:
Flaws in the manufacturing process are a major factor in the development of microcracks in solar panels. Mishandling, choosing less suitable materials, or oversight in quality assurance can introduce weak spots in the panels. These weak points, originating during production, can lead to the start and spread of microcracks. This highlights the importance of detailed attention in manufacturing, thorough training for workers, and strict quality control measures throughout the production process to effectively reduce the likelihood of microcrack formation.
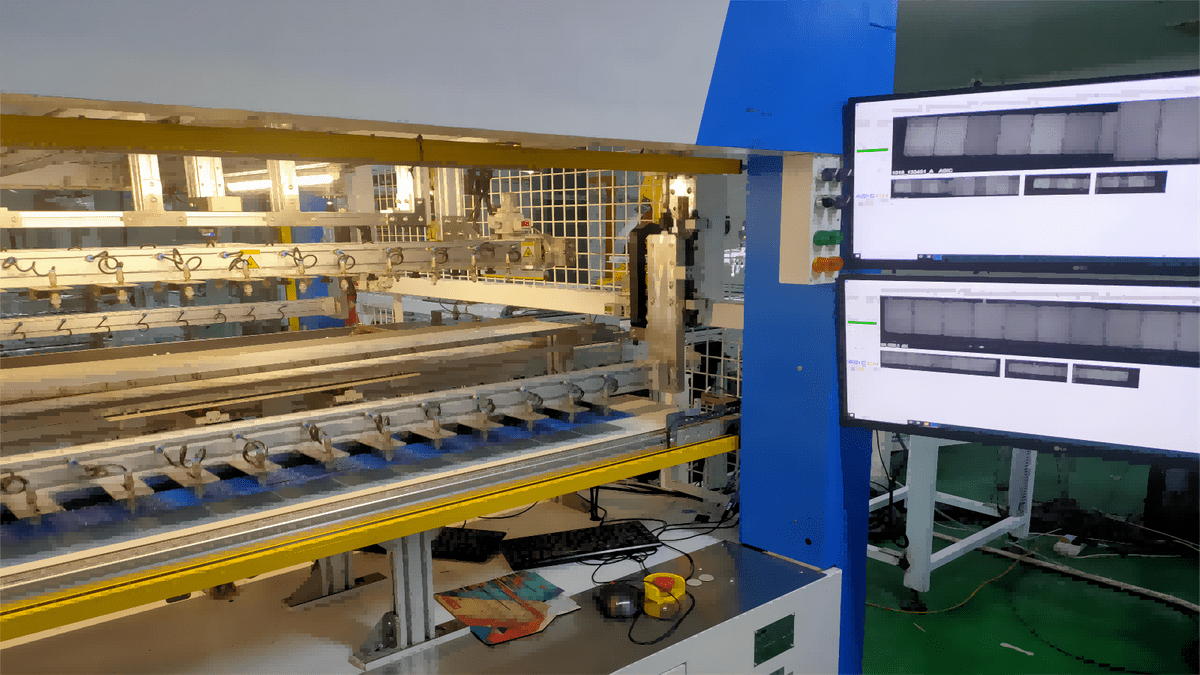
Mechanical stress:
Mechanical stress plays a crucial role in causing microcracks in solar panels, and its impact can be observed during various stages such as transportation, installation, and exposure to external environmental elements.
Throughout the transportation process, solar panels are particularly susceptible to mechanical stress, especially in terms of how they are packed, stored, and handled within the supply chain. Inadequate packing or storing methods can exert undue pressure on the panels, leading to the development of microcracks. Similarly, improper methods of transportation, like rough handling or incorrect stacking, can expose the panels to vibrations and shocks, thereby escalating the risk of microcrack emergence.
Consequently, it's essential to address the challenges associated with packing, storing, and handling in order to effectively reduce the risk of microcrack formation during the transportation of solar panels.
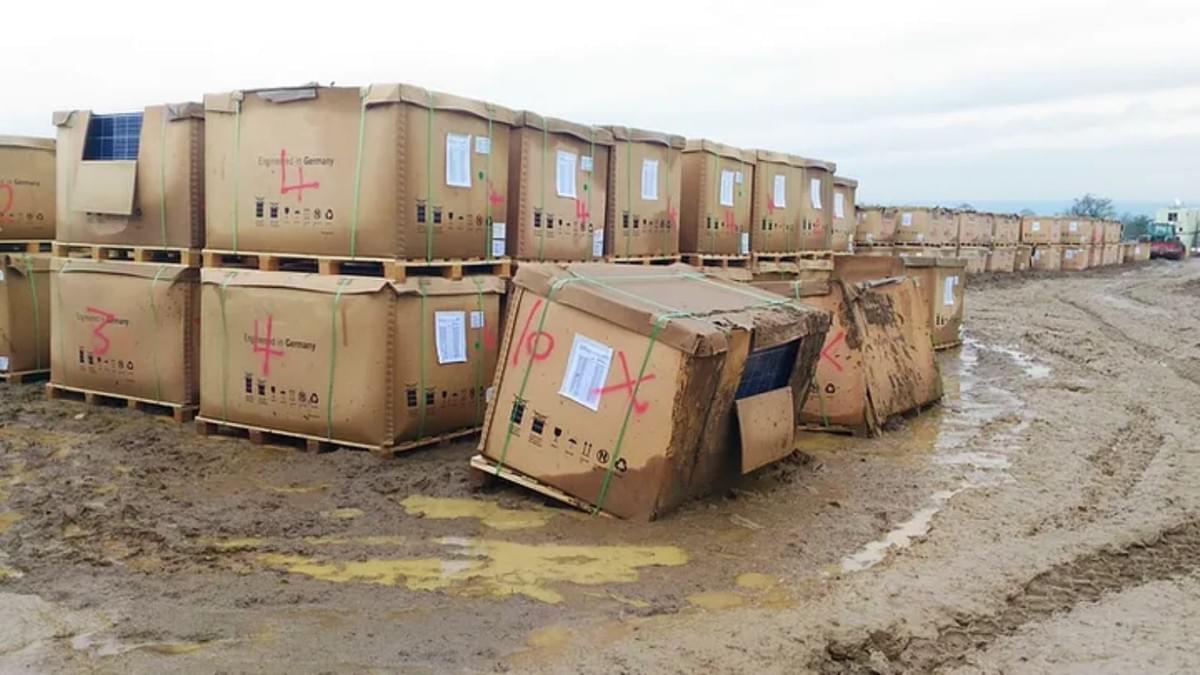
Installation-related mechanical stress is another major factor affecting the integrity of solar panels. Inappropriate practices such as incorrect stacking, placing equipment on the panels, accidental impacts, and setting up on uneven surfaces can create concentrated pressure points that lead to microcracks. Friction-related stress arises when panels are dragged during transportation, and improper handling techniques, such as single-person lifts or resting on the edge of a module, result in disproportionate force distribution. These practices jeopardize the panels' efficiency and durability, underscoring the importance of meticulous handling and installation techniques to reduce mechanical stress and maintain optimal performance.
Experimental findings illustrate this point. For instance, tests showed that resting one's shoulders and head on a panel while climbing stairs caused a 1% decrease in power output. The recommended method for carrying a panel is to evenly distribute its weight by holding it along the frame with both hands.
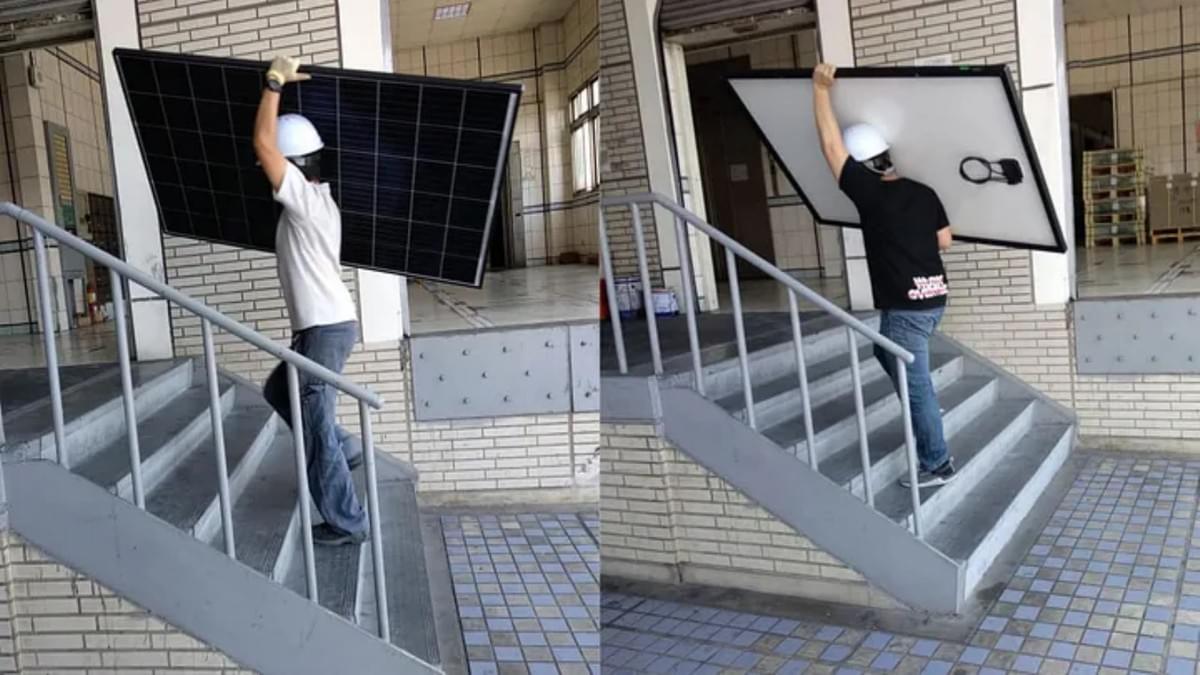
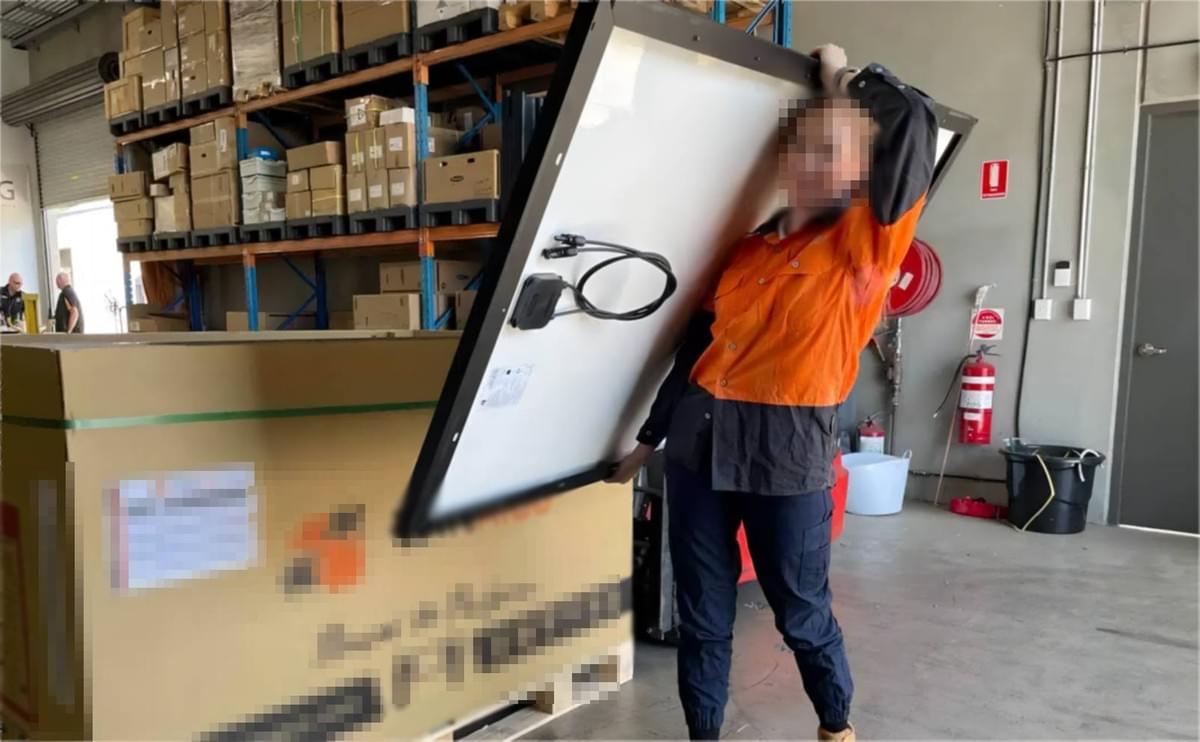
During the extended use of solar panels, external environmental elements, particularly weather-induced stresses like wind, hail, and severe temperature fluctuations, significantly contribute to mechanical stress. These environmental pressures, when sustained over time, lead to the gradual accumulation of microcracks. Thus, a holistic strategy to reduce the risk of microcrack formation in solar panels requires addressing mechanical stress at various phases, including transportation, installation, and operation. By focusing on both internal handling practices and external environmental influences, the overall dependability and efficiency of solar panels can be improved.
Thermal Cycling:
Thermal cycling, caused by daily and seasonal temperature changes, is a key factor in the development of microcracks in solar panels. The continuous expansion and contraction of the materials within the panels, driven by varying sunlight and weather conditions, create an environment conducive to the formation and growth of microcracks over time. The stress from this repeated thermal cycling highlights the need to factor in temperature variations in the design, production, and installation of solar panels, with the aim of increasing their resilience and life span.
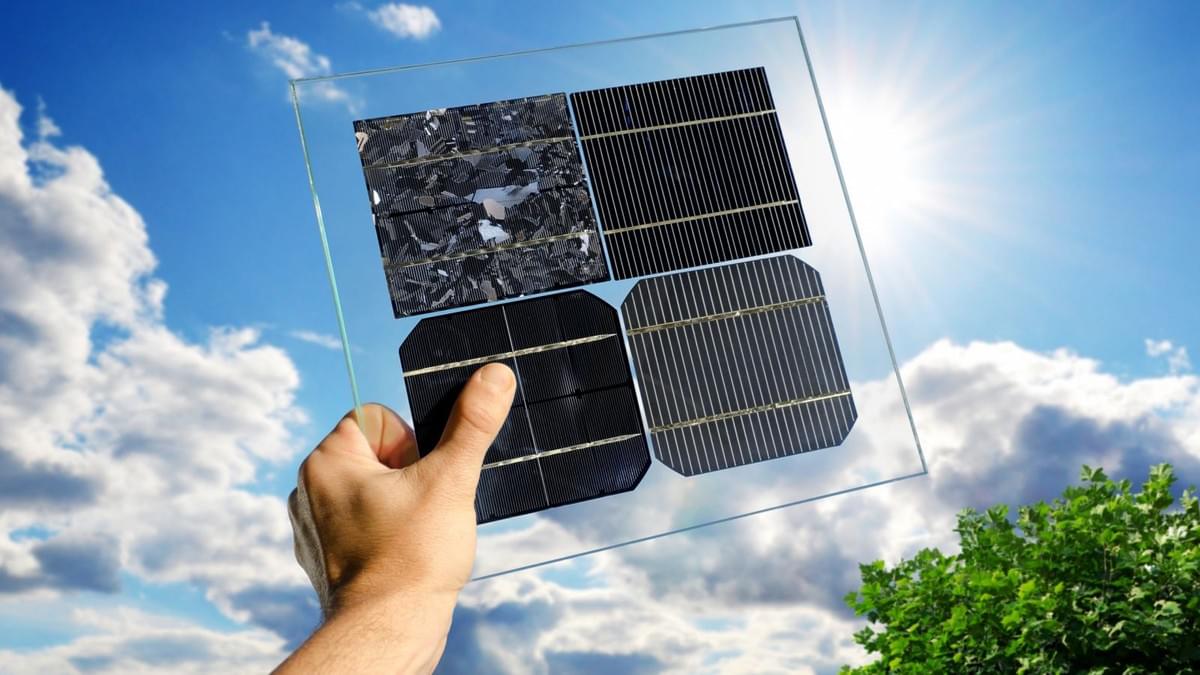
What Damage Can Be Caused by Microcracks?
Increased Resistance and Thermal Damage: Microcracks can lead to an increase in electrical resistance, causing higher temperatures and the formation of hotspots. This enhanced thermal damage further accelerates the deterioration of the impacted solar cell. In some cases, microcracks can expand, cutting off a substantial portion of a cell from the circuit. Such isolation can lead to reverse bias conditions, resulting in even higher temperatures in the connected area of the cell. The rise in temperature poses a threat of permanent damage, reducing energy output and, in extreme situations, may lead to severe outcomes like glass breakage or, rarely, fire due to the intense heat from a cell in reverse bias.
Reduced Power Output: The elevated electrical resistance results in decreased power production, leading to a drop in the overall efficiency of the solar panel.
Crack Propagation and Module Failure: As microcracks grow, they can turn into larger cracks, potentially ceasing or completely stopping the energy production of the entire module. This severe impact requires replacement and significantly shortens the lifespan of the solar panel.
To prevent these harmful effects, it's vital to detect microcracks early through consistent inspections. Various methods, as previously described, are available for early identification and management of microcracks, ensuring the continuous performance and durability of solar panels.
How to Inspect for Microcracks During the Installation and Operation of Solar Panels?
Identifying microcracks in solar panels is crucial for maintaining optimal functionality and extending their lifespan. There are several inspection techniques suitable for different situations during installation and operational phases:
EL (Electroluminescence):
Electroluminescence (EL) is based on the concept of emitting light when an electrical current passes through a material. In solar cells, EL is used to detect and pinpoint defects that are not readily visible through other methods. EL testing is particularly effective in identifying microcracks, broken cells, and other internal imperfections within solar cells. This technique provides a clear view of the cell's structural integrity, revealing hidden problems that might be overlooked by other testing methods.
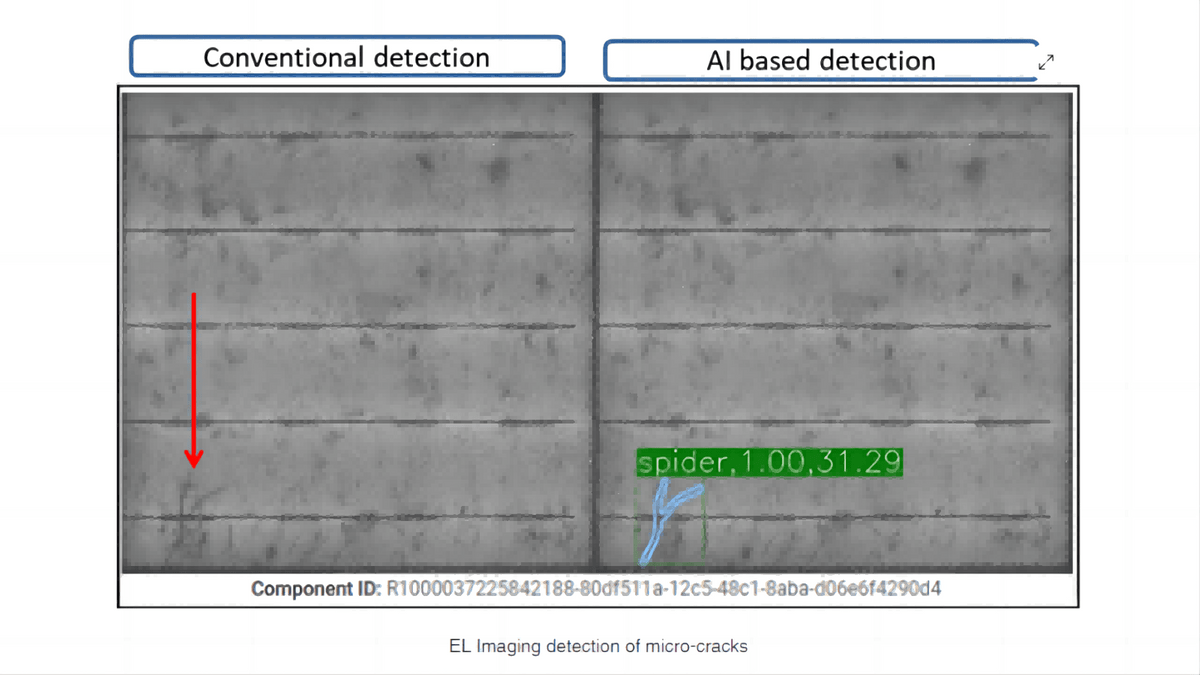
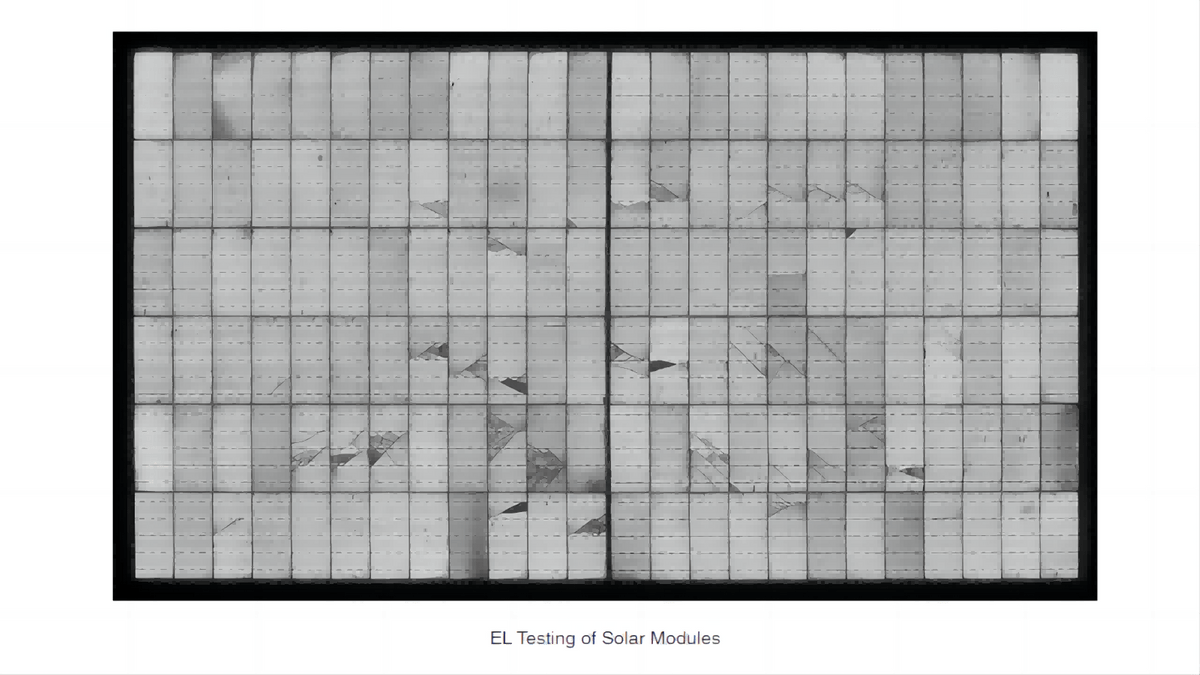
ELCD (Electroluminescence Crack Detection):
ELCD harnesses electroluminescence to effectively detect microcracks in solar panels. By applying an electric current, electroluminescent materials are activated, emitting light that changes in the presence of microcracks, thus making them visible. This non-invasive technique is invaluable for quality assurance in manufacturing and for preventative maintenance in operational settings. ELCD, distinct from standard Electroluminescence (EL), is specifically engineered for microcrack identification, offering superior accuracy in locating and visualizing these small structural faults. The precision of ELCD renders it an essential tool in ensuring the reliability and performance of solar panel installations by identifying issues that might be missed by other testing methods.
Additional Inspection Methods Include:
Photoluminescence Imaging:
Ideal for use during installation and operation, photoluminescence imaging helps detect microcracks that impact the efficiency of photovoltaic conversion. This method can be easily integrated into regular maintenance schedules to maintain continuous efficiency.
Infrared Imaging:
Infrared imaging (IR) works by capturing the thermal emissions from objects, enabling the detection of temperature disparities that signify defects or anomalies. In solar cells, IR is extensively used for thermal evaluations to pinpoint overheating, identify malfunctioning parts, and detect anomalies in solar cells and photovoltaic modules. This technique is particularly adept at finding issues related to electrical connections, such as defective or loose wiring.
Visual Inspection and Microscopic Examination:
Most effective during installation and regular maintenance, visual inspection and microscopic examination involve direct observation and in-depth structural analysis. This direct approach is efficient in identifying visible cracks on the surfaces of solar panels.
In practice, these inspection methods may be used individually or in combination, depending on the specific needs and conditions of the solar panel system. Employing trained professionals is crucial, particularly when using advanced technical equipment, to ensure accurate detection and assessment of microcracks. Consistent monitoring and a thorough inspection strategy are vital for the overall dependability and lifespan of the solar energy system.
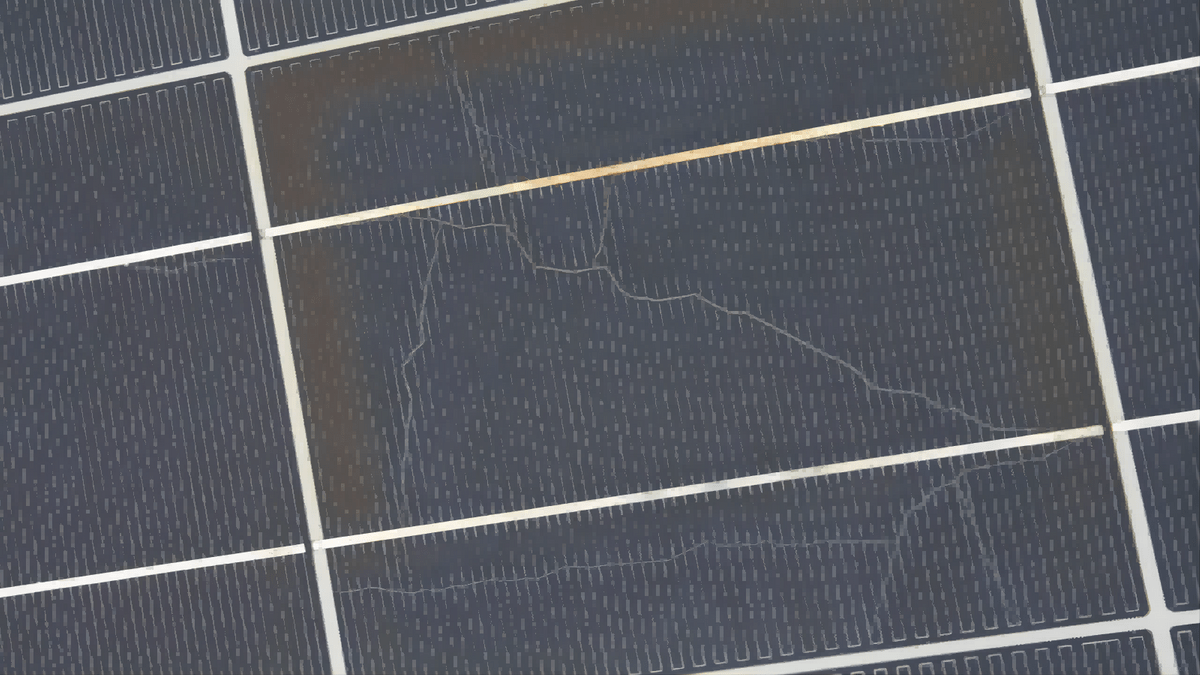
Choose the Right Solar Panels To Reduce Microcracks
In solar panel technology, managing microcracks is essential for maintaining system reliability and performance. Although microcracks are a common issue, recent advancements in technologies like IBC (Interdigitated Back Contact) and HJT (Heterojunction Technology) have greatly diminished their impact on solar panel efficiency. The resistance of these technologies to microcrack formation is outlined in the following points:
HJT Solar Panels:
Type Monocrystalline Silicon Substrate:
HJT solar cells use an N-type monocrystalline silicon substrate, which is highly pure and uniform. This monocrystalline structure, as opposed to the polycrystalline form, is denser and more consistent, contributing to the reduction of microcracks that may arise from stress concentration.
Undoped Amorphous Silicon Layer (i-a-Si:H):
On top of the monocrystalline silicon substrate, a layer of undoped amorphous silicon is applied, serving as a buffer. This layer adds extra flexibility and safeguards the cell during physical stresses such as bending or compression encountered during transportation and installation. This buffering effect helps to mitigate stress on the substrate, thus lessening the likelihood of microcrack formation.
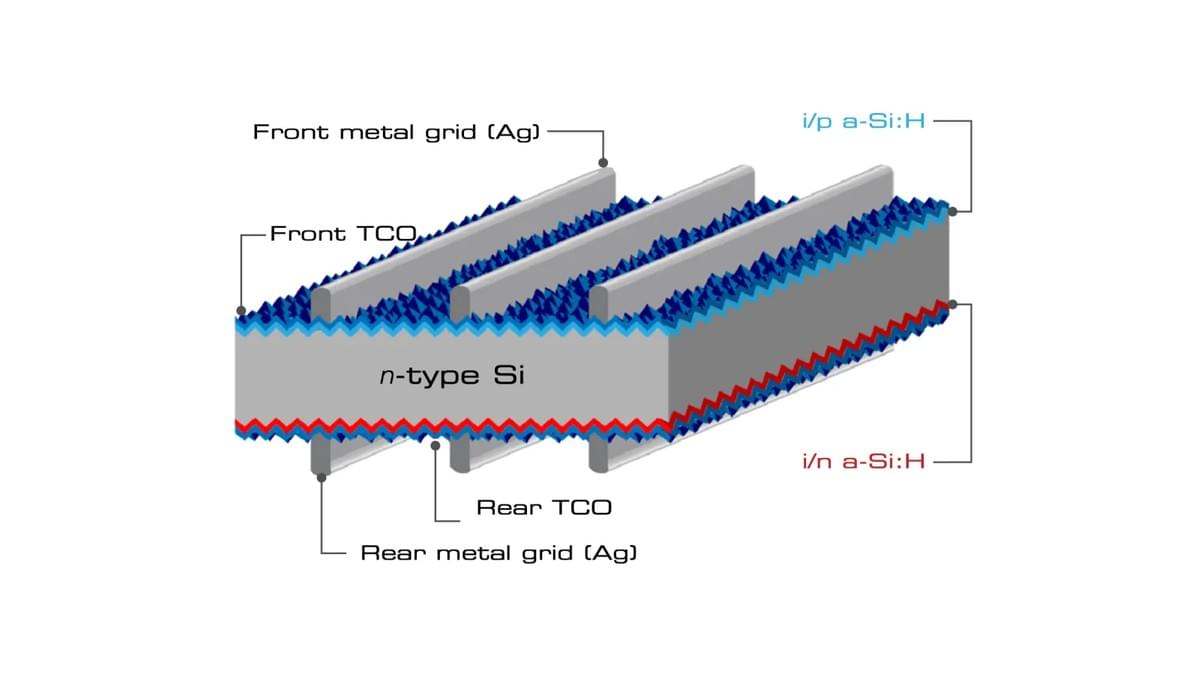
Complete Half-Cell Processing Method:
HJT solar panels incorporate a novel full half-cell processing technique, which completely eliminates cutting damage to the solar cells. This state-of-the-art method greatly reduces the incidence and impact of microcracks on solar panels, proactively addressing potential issues during the manufacturing and handling stages.
Lower Manufacturing Temperature:
HJT cells are produced at lower temperatures compared to traditional crystalline silicon solar cells. This decrease in processing temperature means the materials experience less thermal stress during production, which contributes to the reduction of microcrack formation.
Optimized Overall Structure:
The overall structure of HJT cells is carefully optimized during the design and manufacturing process to boost their resistance to physical stress. For example, the design includes smoother edges and corners of the cells, which helps in minimizing stress concentration points.
Ultra-Multi Busbar Design:
HJT panels are designed with an ultra-multi busbar system featuring narrower busbars. This design reduces the amount of silver paste used, which in turn decreases shading effects and shortens the distances for current transmission. Not only does this improve the electrical performance of the panels, but it also lessens the impact of current loss caused by microcracks, damaged busbars, and fractures. This enhancement in design contributes to the overall reliability and extended lifespan of the panels.
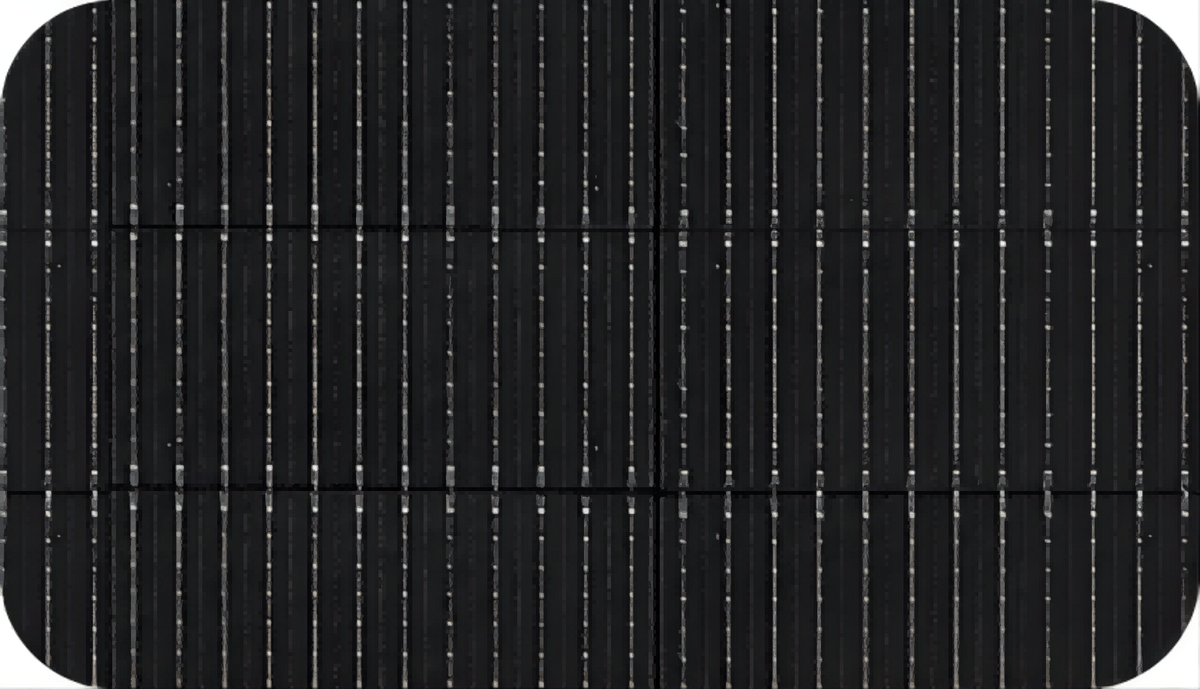
Enhanced Mechanical Strength:
The combination of the design and manufacturing improvements previously described significantly bolsters the mechanical strength of HJT solar cells. This enhancement in strength is crucial for preserving the integrity of the cells during transportation and installation processes, thereby diminishing the likelihood of microcrack formation.
Maysun Solar capitalizes on the exceptional benefits of heterojunction technology (HJT), leading to a remarkable evolution in their HJT solar panels. These panels represent a major step forward in solar power generation, not only achieving a marked increase in efficiency but also demonstrating a significant enhancement in their resistance to microcracks. This dual advantage of superior efficiency and durability positions Maysun Solar's HJT panels as a leading choice in solar energy solutions.
IBC solar panels from Maysun Solar offer a notable advantage in weather resistance. Their All Back Contact design effectively prevents tensile damage and detachment caused by the thermal expansion and contraction of the front welding strip under extreme temperature stress. This feature ensures consistent and stable power generation, reducing the negative effects of thermal fluctuations on the integrity of the solar panels.
Maysun Solar's latest generation of interdigitated Back Contact (IBC) solar panels is a blend of resistance to microcracks, high efficiency, aesthetic appeal, and an extended lifespan. Choosing these IBC solar panels is a prudent decision for those looking to enhance both efficiency and performance in their solar energy systems. The IBC 430W Full Black solar panels, installed on a roof by a German customer of Maysun Solar, demonstrate this combination of advanced technology and design. Further information is available for those interested in exploring these innovative solar panels.
Maysun Solar has been specialising in producing high quality photovoltaic modules since 2008. Choose from our wide variety of full black, black frame, silver, and glass-glass solar panels that utilise half-cut, MBB, IBC, HJT and Shingled technologies. These panels offer superior performance and stylish designs that seamlessly blend in with any building. Maysun Solar successfully established offices, warehouses, and long-term relationships with excellent installers in numerous countries! Please contact us for the latest module quotations or any PV-related inquiries. We are excited to assist you.
Reference:
Chang, T. (2023, July 31). How microcracks damage solar panels – WINAICO Australia. WINAICO Australia. https://www.winaico.com.au/blog/what-cause-microcracks
RenewSys India. (2023, February 3). Micro cracks in solar modules: Causes, detection and prevention. renewsysworld. https://www.renewsysworld.com/post/micro-cracks-in-solar-modules-causes-detection-and-prevention
You may also read: