Contents:
1.Why Cut Solar Cells?
2.The Principles of Cutting
3.Advantages of 1/3 Cut Panels over Half-Cut Panels
4.Why Don't Manufacturers Produce 1/4-Cut or Even 1/5-Cut Solar Cells?
5.Conclusion
Why Cut Solar Cells?
In recent years, photovoltaic (PV) technology has rapidly advanced and become widely used. The demand for high-power solar panels is increasing, and reducing energy loss while boosting the output power of these panels has become a focus for manufacturers worldwide. Cutting solar cells is a technique used to enhance panel efficiency by making the cells smaller, which reduces resistance and improves power output.
But why has cutting solar cells only recently become a popular topic in the industry? One reason is the increase in the size of silicon wafers from 156mm (M1) to 161.7mm (M4). This size increase has boosted the wafer area and current by about 7%, but it also increased electrical losses by 15%. This spurred the industry to find ways to reduce current-related losses. Additionally, cutting cells can reduce shading losses from the cell's metal electrodes and increase the number of busbars, which helps improve current flow.
Moreover, advancements in wafer and cell manufacturing processes now allow for the screening of full-size cells without the need to re-measure cut cells after they are divided. This streamlines the production process, making it more efficient and cost-effective.
In summary, cutting solar cells into smaller pieces helps make solar panels more powerful and efficient, meeting the growing demand for high-performance solar energy solutions.
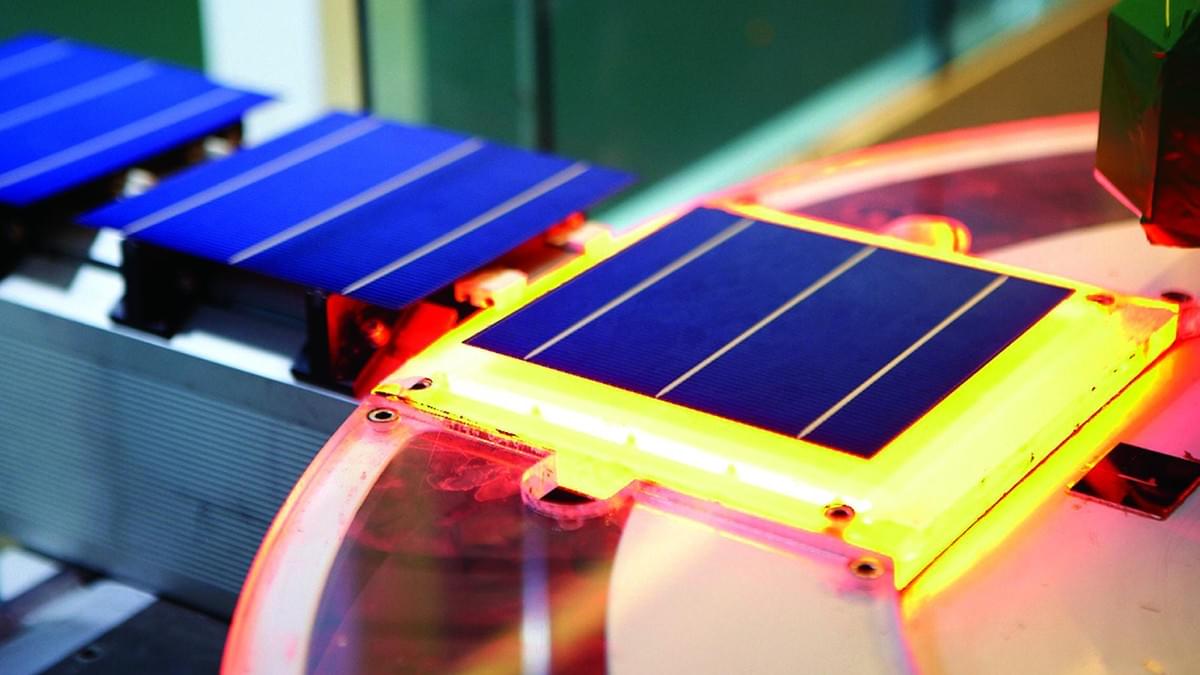
Principles of Cutting Solar Cells
1. Cutting Process
- Squaring the Silicon Ingot: Processing the silicon ingot into a block that meets required specifications.
- Silicon Block Cutting and Grinding: Removing the ends and flattening, chamfering, and rounding the silicon block.
- Silicon Block Gluing: Bonding the silicon block to a workpiece plate in preparation for wire cutting.
- Silicon Block Cutting: Using a multi-wire saw to cut the silicon block into thin silicon wafers.
- Silicon Wafer Cleaning: Cleaning the wafer surface of slurry through pre-cleaning, inserting, and ultrasonic cleaning.
- Silicon Wafer Sorting and Packaging: Grading the wafers according to standards and packaging them for storage.
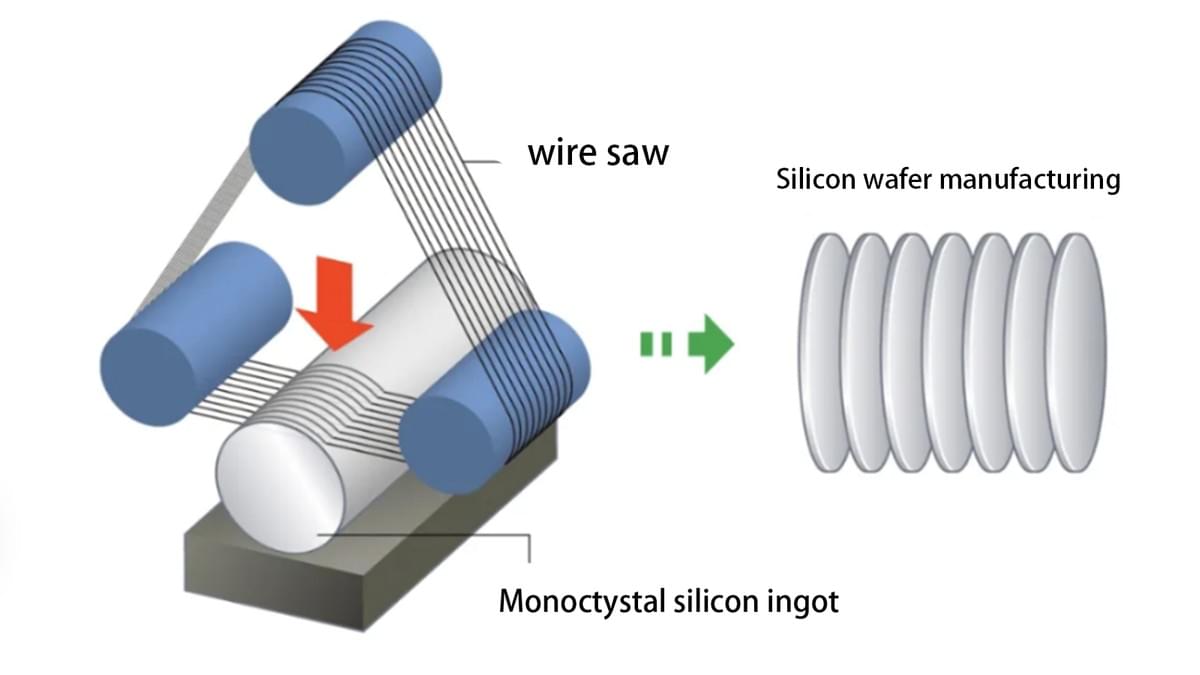
2. Cutting Techniques
LSC - Laser Scribing and Cleaving
This technique relies on laser ablation technology. Half-cut cell technology typically employs laser cutting, where standard-sized solar cells are sliced vertically along the main busbars into two equal halves. These halves are then interconnected through welding for series connection.Here’s how it works:
Process: A laser creates full-length scribe lines along the edges of the half-cut cell. In some cases, the scribing doesn’t fully separate the cell but leaves a groove about half the cell’s thickness. The cell is then mechanically broken along these scribe lines.
Advantages: This method avoids creating shunt pathways in the p-n junction by performing the scribing from the back of the cell. For Passivated Emitter and Rear Contact (PERC) cells with a complete rear metal layer, creating a small opening on the back doesn’t cause any power loss.
Innovations: Fraunhofer CSP has developed and patented an advanced version of the LSC technique. This involves applying laser scribing to slightly bent solar cells, achieving a one-step process where scribing and breaking occur in the same station.
TMC - Thermal Mechanical Cleaving
Unlike LSC, TMC doesn’t use ablation techniques that can cause microcracks. Instead, it applies a highly concentrated thermal gradient along the edge of the half-cut cell, inducing localized mechanical stress that results in cracking.
Process: By applying a thermal gradient, the material undergoes local mechanical stress that leads to cracking without ablating the material.
Advantages: TMC processes don’t involve ablation and reduce overall thermal side effects, which minimizes structural damage to the wafers when process parameters are optimized.
Innovation: Some equipment for TMC half-cut cells is already commercially available or in development. Notable manufacturers include 3D-Micromac AG and Innolas Solutions GmbH from Germany.
In summary, cutting solar cells involves a series of precise steps to ensure optimal performance and efficiency. Both LSC and TMC techniques offer different advantages and can be chosen based on specific needs and manufacturing capabilities.
Advantages of 1/3-Cut Cells Compared to Half-Cut Cells
1. Reduced Resistance Losses and Increased Output Power
One source of power loss in solar panels is resistance loss, which occurs during current transmission. Solar cells use busbars to connect to adjacent wires and cells, and the current flowing through these busbars causes some energy loss. By cutting solar cells into halves, the current produced by each cell is halved, resulting in lower resistance losses as the current flows through the cells and wires of the solar panel.
Using the formula for electric power loss P=I2RP = I^2RP=I2R, when the current is reduced to one-third of its original value, the power loss is significantly reduced. With 1/3-cut cells, the current is only one-third of that of the original cell, compared to half in half-cut cells. This further reduces the series resistance of the cells, minimizing energy loss and thereby increasing the output power and efficiency of the solar modules.
2. Reduced Hot Spot Effect
In traditional full-size cell modules, if a cell is shaded, it can create a hot spot, leading to performance degradation or even damage to the cell. The 1/3-cut cell technology reduces the risk of hot spots by increasing the number of cells and thereby lowering the current in each cell. With a more even heat distribution and reduced hot spot effects, 1/3-cut modules have an extended lifespan and higher long-term reliability.
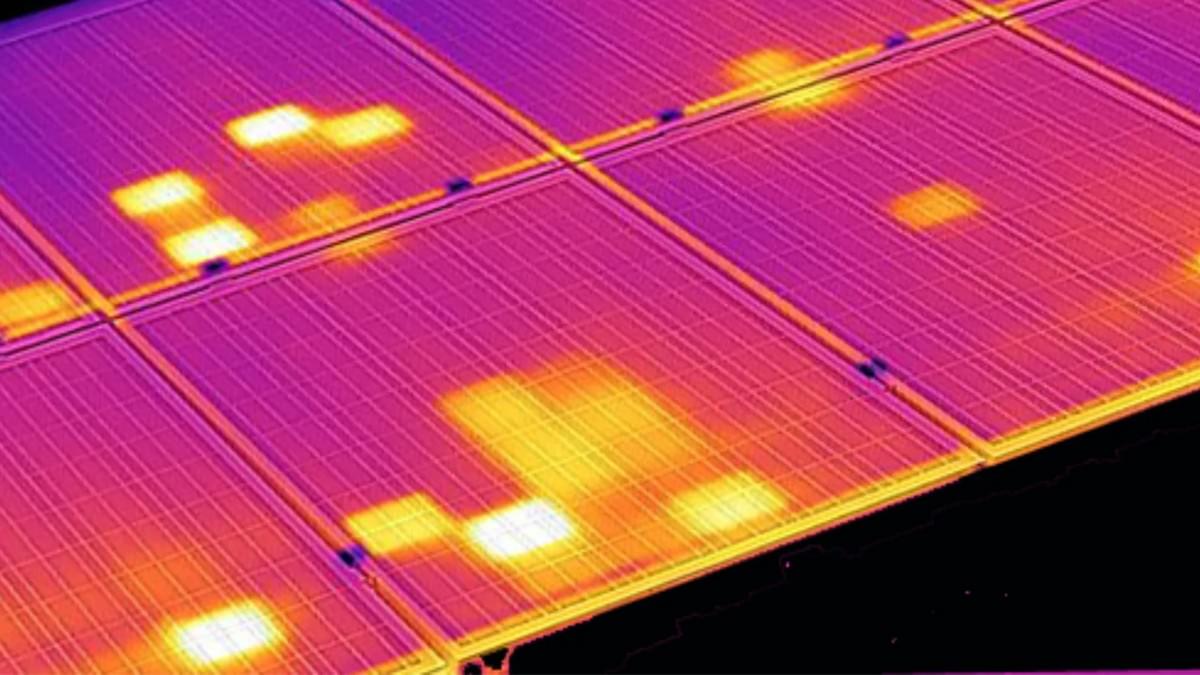
3. Increased Fill Factor
Fill factor (FF) is a measure of quality of a solar cell. This is the available power at the maximum power point (Pm) divided by the open circuit voltage (VOC) and the short circuit current (ISC):

The fill factor is directly affected by the values of the cell's series, shunt resistances and diodes losses. Increasing the shunt resistance (Rsh) and decreasing the series resistance (Rs) lead to a higher fill factor, thus resulting in greater efficiency, and bringing the cell's output power closer to its theoretical maximum.
The 1/3-cut cell technology improves current management, enhances the module's fill factor, and thus performs better under real operating conditions.
4. Improved Shadow Tolerance
Compared to full-size cells, half-cut cells demonstrate greater resistance to shadow effects. This is not due to the cells themselves, but rather to the wiring method used to connect the half-cut cells within the panel. In traditional solar panels constructed with full-size cells, cells are connected in series, where shading of one cell in a series can stop the entire row from generating power. A standard panel typically has 3 rows of independently connected cells, so shading one cell in a row eliminates half of that panel's power output.
Similarly, half-cut cells are also connected in series, but panels made with half-cut cells have twice the number of cells (120 instead of 60), resulting in twice the number of independent cell rows. This wiring configuration reduces power loss in panels built with half-cut cells when a single cell is shaded, as shading one cell can only eliminate one-sixth of the panel's total power output.
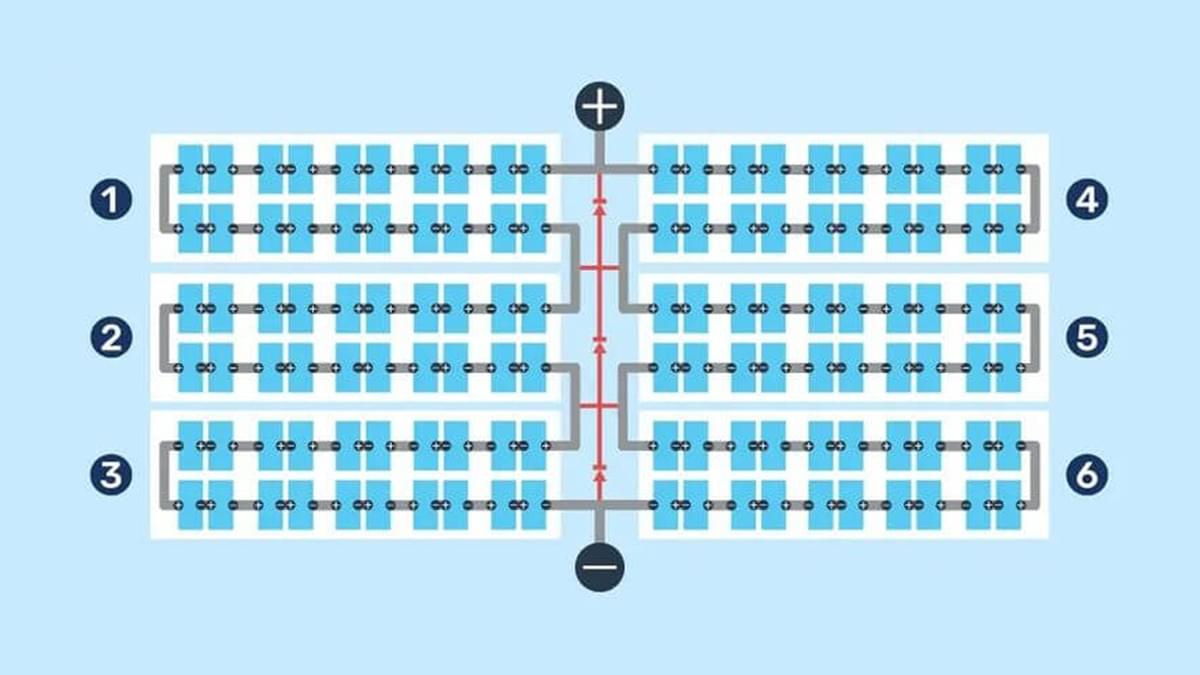
By extension, 1/3-cut cells exhibit even lower sensitivity to local shading compared to half-cut cells. Even if some cell segments are shaded, the overall power output remains largely unaffected, ensuring higher overall efficiency in electricity generation.
5. Enhanced Market Competitiveness
The improved performance and efficiency of 1/3-cut modules make them more competitive in the market, capable of meeting the demands of high-end markets and specialized applications. This enhancement reduces expenditure costs, thereby yielding higher economic benefits.
With increased power output per module, fewer modules are needed to generate the required electricity for both ground-mounted and rooftop solar installations. This reduction in module quantity contributes to minimizing the space required for installation. For large utility-scale solar farms, the smaller space requirement helps in reducing the land needed to establish solar photovoltaic facilities. This, in turn, lowers capital expenditure costs for solar developers, as land represents a significant initial investment for constructing large-scale solar power plants.
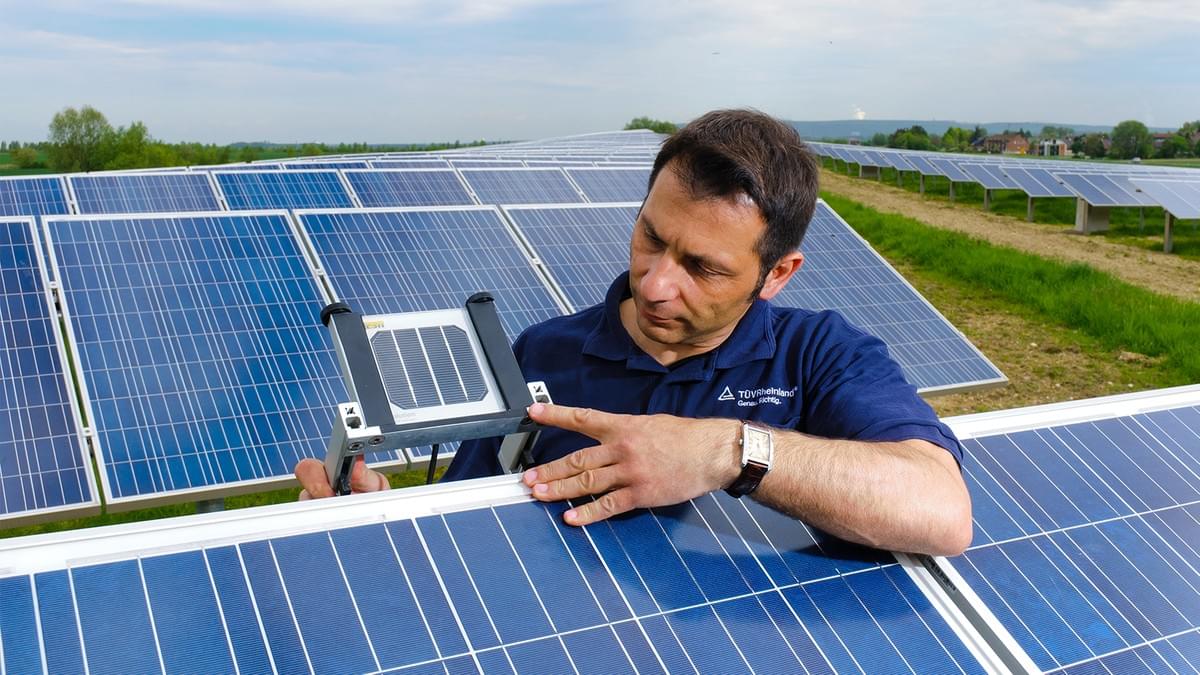
Why Don't Manufacturers Produce 1/4-Cut or Even 1/5-Cut Solar Cells?
While 1/4-cut and 1/5-cut modules could offer slightly higher power output per module, optimizing an energy solution involves considering additional manufacturing complexities.
Specifically, incorporating more solar cell cuts necessitates additional bypass diodes for circuit protection within the module. This increases the use of raw materials, resulting in added costs and extended production timelines. For residential solar solutions focused on cost reduction alongside efficiency, keeping modules sleek and straightforward is advantageous. 1/3-cut solar cells, requiring only three bypass diodes, strike a balance between affordability and enhanced performance for end users. This design also minimizes risks associated with future upgrades while maximizing current efficiency capabilities.
Conclusion
1/3-cut solar cells, compared to half-cut cells, significantly enhance the overall performance and efficiency of solar panel modules by further reducing current and resistance, minimizing power losses, optimizing heat distribution, and improving component reliability. These advantages make 1/3-cut technology more appealing for high-end applications and specific scenarios. Despite the more complex manufacturing process, the performance improvements and economic benefits often outweigh these additional costs.
Maysun Solar has been specialising in producing high quality photovoltaic modules since 2008. In addition to the Balcony Solar Power Station, Maysun Solar offers wide variety of full black, black frame, silver, and glass-glass solar panels that utilise half-cut, MBB, IBC, and HJT technologies. These panels offer superior performance and stylish designs that seamlessly blend in with any building. Maysun Solar successfully established offices, warehouses, and long-term relationships with excellent installers in numerous countries! Please contact us for the latest module quotations or any PV-related inquiries. We are excited to assist you.
References:
Sharma, N. (2024, March 15). Half-Cut Solar Cells- Are they Worth the Hype? Ornate Solar. https://ornatesolar.com/blog/why-should-you-choose-half-cut-cell-modules-for-your-solar-projects
Trina Solar. (2022, October 31). What’s the big hype about 1⁄3-Cut solar cells? https://www.trinasolar.com/us/resources/blog/third-cut-solar-cells
Wikipedia contributors. (2024, April 21). Solar-cell efficiency. Wikipedia. https://en.wikipedia.org/wiki/Solar-cell_efficiency
You may also like: